Application of high pressure for structure modification of food and food ingredients
DLG Expert report 6-2018
Author:
- Dr.-Ing. Kemal Aganovic, Head of Advanced Technologies, Deutsches Institut für Lebensmitteltechnik e.V., Prof.-von-Klitzing Str. 7, Quakenbrück, Germany, k.aganovic@dil-ev.de
Contact:
- Carola K. Herbst, Project Manager, Competence Center Food, DLG e.V., C.Herbst@DLG.org
High pressure homogenization for production of low fat emulsions
Initial situation
Homogenization is a mechanical process for reducing the relative heterogeneity of a system and for producing a homogeneous size distribution of particles suspended in a liquid. A high-pressure homogenization (HPH), also known as dynamic or ultra-high pressure homogenization (UHPH), is a relatively new technology recently introduced through the development of a new generation of homogenizers. This new generation is capable of attaining pressures 10 – 15 times higher than traditional homogenizers, opening a wide range of new possibilities in food processing and biotechnology. In early 1980s a new technology was introduced for production of fine emulsions based on the availability of devices able to generate and manage very high pressures in liquids as high as 4000 bar, as well as on a availability of new homogenization valve design [1]. The key difference compared to traditional homogenizers is seen in a new, narrow disruption valve design. The gap of the UHPH valve is significantly smaller (2-5 µm) compared to the valve gap of a traditional homogenizer (10-30 µm) [2]. Different manufacturers of high-pressure homogenizers exist nowadays, producing either prototype or industrial scale equipment, such as AVP, Microfluidics, Stansted Fluid Power, AVP, Avestin, BEE International, GEA, Niro Soavi and other companies.
Area of application
Depending on the purpose of the HPH treatment, two main areas can be identified. The first area is mainly concerned with the physical changes induced through HPH processing. These changes are seen in reduction of particle size and narrowing the size distribution of particles, droplets, micelles, in a suspension or an emulsion; in ability for stabilization of emulsions or preparation of nanoparticles and nano-suspensions, as well as for attaining viscosity and texture changes. The second area is focused on the effect of cell disruption induced at the homogenization valve, which can be either applied for recovery of intracellular material in biotechnology and pharmaceutical industry, or applied to reduce the microbial load in food, pharmaceutical and similar liquid and semi-liquid products.
Homogenization technique and occurring phenomena
Current industrial, pilot-scale or lab-scale homogenizers are equipped with a high-pressure pump, such as a positive-displacement pump coupled with a pressure intensifier that forces the process fluid through a specifically designed valve or a nozzle, which are blocking the forced liquid and thus generate the pressure. The processed liquid passes under high pressure through a convergent section (homogenization gap) and then expands. In systems with needle and seat, the pressure can be regulated by adjusting the gap between. The nozzle was developed for water-jet cutting applications, where homogenization is achieved by nozzle and the pressure is determined by the high pressure pump [3]. Needle, seat and nozzle should be made of a resistant material, such as ceramic, zirconium, tungsten carbide or diamond, with the letter one being the most resistant, but also the most expensive option. Due to pressurization of the process fluid (up to 350 MPa) the temperature will increase in the order of 3°C per 100 MPa due to adiabatic heating. Sudden pressure release results in a significant temperature increase caused by shearing and partial conversion of dynamic energy into heat in the range of 15-20 °C/100 MPa (for aqueous matrices). Most pilot and industrial scale homogenizers are equipped with two stages of homogenization valves. The first stage is allowing for attaining high pressure range and the second stage is in function of a back pressure of the first one, reducing the cavitation and allowing for less-disturbed operation and less pressure fluctuation. Due to high temperatures that may occur at high pressure levels, very often the product is cooled down between the first and the second stage valve to reduce the thermal load on the heat sensitive products. In total the fluid is exposed to high pressures very short time (1-10 s), between the intensifier and the valve.
During the homogenization, there are several phenomena occurring at the valve, such as pressure, shear stress, cavitation, impingement and temperature increase, and the HPH treatment effects cannot be attributed to a single phenomenon. It is rather a combination of all that have impact on cell disruption, food constituents and molecules. However, the major parameters determining efficiency of the process are operating pressure, temperature, homogenizer valve design and number of passes through the valve. Moreover inlet temperature, viscosity of the product and maximum particle size are crucial for successful operation of homogenizer.
Effect of HPH on emulsions
HPH is a continuous technique for preparation and stabilisation of emulsions, by means of significantly reducing the size of oil droplets and improving interactions between emulsifier and fat phase. This allows for production of oil-in-water (o/w) emulsions with reduced fat content. Further, emulsion with reduced amount of emulsifiers or completely free of thickeners can be produced. Thus a mayonnaise-like product with as low as 30% oil, and containing liquid egg yolk as a natural emulsifier, and other natural ingredients such as salt, sugar, mustard, vinegar and citric acid, without additional emulsifiers and thickeners can be produced. Such HPH produced emulsion has similar rheological properties to a full fat commercial mayonnaise. By comparing the results of the oil droplet size distribution, it can be clearly seen that UHPH yielded significantly smaller oil droplets compared to the commercial full (CFFM) and low fat emulsion (CLFM), indicated by a shift of the droplet size distribution curves in the direction of smaller particles for the UHPH emulsion (Fig. 1). Also microscopic images revealed different structures obtained in UHPH emulsions compared to CFFM and CLFM (Fig. 2). In case of full fat emulsion it can be seen that the fat (coloured red) is predominant phase, compared to low fat emulsion. However, in the UHPH a more even distribution of fat droplets and protein (emulsifier) (on images coloured green) was achieved [4].
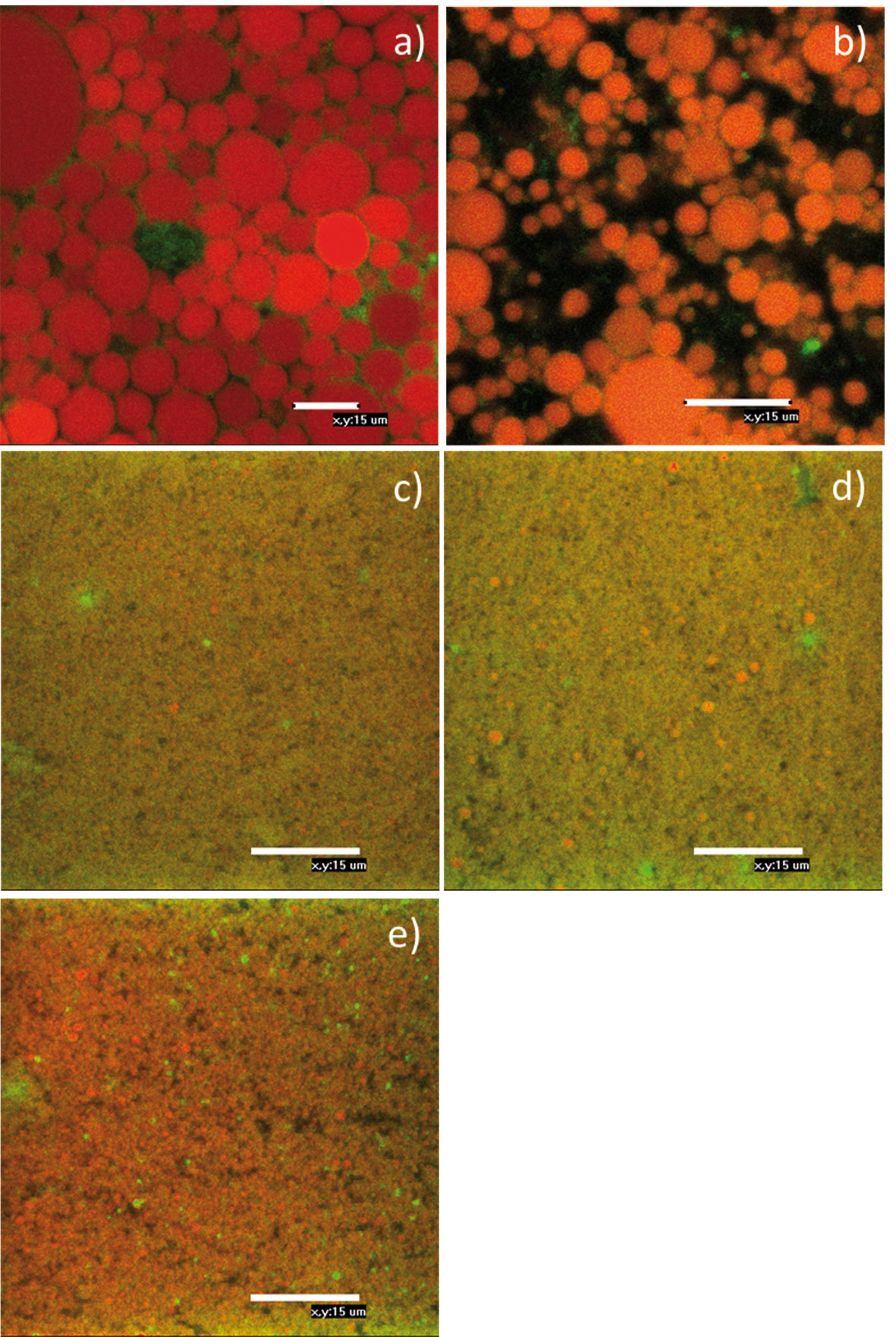
(a) commercial full fat mayonnaise (CFFM), (b) commercial low fat mayonnaise (CLFM) and emulsion produced by UHPH at (c) 100 MPa, (d) 200 MPa and (e) 300 MPa. Proteins were coloured in green and fat globules were coloured in red
Modification of hydrocolloids through static and dynamic high pressure
Initial situation
Human diseases are often linked with malnutrition, coming from low vitamin intake, high consumption of salt, fat and sugar. A large part of the human salt intake is coming directly from the processed food, mostly meat. High salt consumption (8 - 10 g/day) in industrialized countries is linked to high blood pressure and other cardiovascular diseases [5]. Beside its use as a flavour enhancer, salt is used in the food industry for preservation and it has crucial function in structure formation, interacting directly with food constituents, for example proteins. Product texture and juiciness are meat quality parameters and are closely linked to the salt content due to impact of salt presence on protein hydration.
Principle of action
High pressure processing (HPP) is an alternative technology with potential to deliver products with improved nutritional quality, but also products reduced in salt. Compared to continuous process of UHPH, HPP is a batch process where product is exposed to hydrostatic pressure of up to 6000 bar, with the aim of inactivation of harmful and spoilage bacteria in food products. The pressure has minimal impact on covalent bonds of bioactive compounds. Thus smaller molecules such as vitamins are minimally affected by the treatment. The pressure alters the distance between the molecules having impact on non-covalent bonds (van der Waals forces, hydrogen bonds, hydrophobic interactions etc.) and thus larger molecules e.g. proteins, can be differently affected. High pressure induced modification of proteins involves changes of secondary, tertiary and quaternary structure, from native state via intermediate state to the denatured state. Depending on the pressure level, protein functionality such as solubility, gelation, emulsification, foaming, water binding or water holding capacity can be modified. Pressure levels between 5000-6000 bars are often used for food preservation purposes, but in case of raw meat lead to protein denaturation and solidification, and in case of red meat to colour changes. Pressures in the range of 3000 bars often lead to protein solubilisation and hydration. Lower pressure levels of 1000 bars allow protein conditioning and gelation, leading to similar effect as presence of salt. Within the EU project Pleasure (http://www.pleasure-fp7.com) potential of different technologies to reduce unwanted fat, sugar and salt in different products were investigated with the potential to reduce unwanted fat, sugar and salt in different products. The results indicated that application of pressure is one of the tools for protein conditioning in salt reduced ham or sausage, with similar appearance, textural and sensorial attributes as a reference one (Fig. 3). On the other hand, HPP can be used as an energy efficient and fast “cold-denaturation” step to replace traditional thermal cooking and improve water holding capacity of meat and fish products. As pressure is uniformly distributed throughout the product without delay, the process can be considered as time efficient, resulting in inactivation of microorganisms and a product with less cooking loss.
Structure modification of ingredients
Continuous application of high pressure (high pressure homogenization) has also shown potential for functionality of some hydrocolloids. When solution of carrageen, alginate or similar compound, is exposed to high homogenization pressures of 100-300 MPa, gels with improved water binding capacity, water immobilization and strength can be obtained. It is believed that depolymerisation and conformational changes at the expansion area of the valve take place, which leads to improved functionality. Beside water binding properties and gel strength, the product after the HPH tends to show less syneresis during storage period compared to untreated hydrocolloids [6].
Hydrodynamic shockwave treatment for meat tenderisation
Processing methods for tissue disintegration
Currently, different mechanical, (bio-)chemical and thermal methods are used for disintegration of organic and inorganic materials. In mechanical processing of e.g. rocks or electronic scrap, hydrodynamic processes are used for disintegration and fractioning in addition to conventional crushing and milling [7, 8]. Also in medical application extracorporeal shockwave therapy has been successfully used since the 1980s for destruction of kidney stones and other deposits [8]. In food processing, softening of meat tissue is often desired and achieved through maturing and/or mechanical processing for improvement of tenderness [9, 10]. For mechanical disruption of the muscle tissue, treatment such as high pressure, ultrasound or pulsed
electric fields are suggested [11], while the use of explosive-generated hydrodynamic shockwaves was investigated on an industrial scale in the United States in 1990s [12]. However, due to safety and legal limitations, the process was abandoned. Further development of the technology allowed for a more safe and reproducible hydrodynamic shockwave process. Shockwaves are mechanical pressure pulses that can be produced in gases or liquids. The shockwave can be generated by piezoelectric, electromagnetic, electrothermal, or electrodetonative methods [13]. In case of electrical discharge, the electrical energy is stored in capacitors and discharged via electrodes under water. Meanwhile, two prototype shockwave machines were developed and built for continuous operation for the purposes of meat tenderisation. The system is equipped with a conveyer belt that transports meat cuts into the treatment area under water, where continuous electrical discharges in pulses take place to generate the shockwave. Treatment intensity is directly influenced by the energy storage, current and voltage applied. Number of pulses applied to an individual meat piece depends on the length of the meat piece and the frequency of pulses applied, which is dependent on the belt speed. These parameters allow for an adjustable and targeted treatment of shockwave intensity and number of pulses applied per length.
So far the most of the research activities focused use of shockwave for meat tenderisation, but some other applications were proposed such as opening of oysters, improvement of extraction, softening of plant tissue and production of rice flour. In meat, two major effects were recognised contributing to tenderisation: (1) physical changes in muscle structure and (2) enhanced proteolysis of muscle protein [14]. However, it has been shown that the shockwave treatment did not increase the enzymatic activity, but rather enhanced the contact between structural muscle proteins and endogenous proteolytic enzymes [15].
In general, improvement of tenderness can be achieved in different meats such as beef, pork, lamb or poultry, ranging usually from 10-70%, depending on the meat initial tenderness, animal species and meat cuts, and other parameters.
Literature:
- [1] Donsi F, Ferrari G, Maresca P. High-Pressure Homogenization for Food Sanitization. Global Issues in Food Science and Technology. San Diego: Academic Press; 2009. p. 309-52.
- [2] Floury J, Bellettre J, Legrand J, Desrumaux A. Analysis of a new type of high pressure homogenizer. A study of the flow pattern. Chemical Engineering Science. 2004;59:843-53.
- [3] Harte F. Food Processing by High-Pressure Homogenization. In: Balasubramaniam VM, Barbosa-Cánovas GV, Lelieveld HLM, editors. High Pressure Processing of Food: Principles, Technology and Applications. New York, NY: Springer New York; 2016. p. 123-41.
- [4] Aganovic, Kemal, Ute Bindrich, and Volker Heinz. “Ultra-high pressure homogenisation process for production of reduced fat mayonnaise with similar rheological characteristics as its full fat counterpart.” Innovative Food Science & Emerging Technologies 45 (2018): 208-214.
- [5] Ruusunen M, Puolanne E. Reducing sodium intake from meat products. Meat Science. 2005;70:531-41.
- [6] Albers, D., Aganovic, K., Franke, K. 2016. Verfahren zur Herstellung von Hydrokolloid mit erhöhtem Wasserbindevermögen. EP3254569A1
- [7] Loeffler M. Commercial Pulsed Power Applications in Germany. Symposium on Pulsed Power and Plasma Applications. Kailua-Kona, Hawaii. 2002.
- [8] Bluhm H. Pulsed Power Systems: Springer, Berlin, Heidelberg; 2006.
- [9] Berry BW, Smith GC, Carpenter ZL. Relationships of certain muscle, cartilage and bone traits to tenderness of the beef longissimus. Journal of Food Science. 1974;39:819-24.
- [10] Denoyelle C, Lebihan E. Intramuscular variation in beef tenderness. Meat Sci. 2004;66:241-7.
- [11] Lopp A, Weber H. Untersuchungen zur Optimierung der Zartheit von Rindfleisch. Fleischwirtschaft 2005;85:111-6.
- [12] Solomon MB, Long JB, Eastridge JS. The hydrodyne: a new process to improve beef tenderness. Journal of animal science. 1997;75:1534-7.
- [13] Bolumar T, Toepfl S. 9 - Application of Shockwaves for Meat Tenderization. Innovative Food Processing Technologies: Woodhead Publishing; 2016. p. 231-58.
- [14] Bolumar T, Enneking M, Toepfl S, Heinz V. New developments in shockwave technology intended for meat tenderization: Opportunities and challenges. A review. Meat Science. 2013;95:931-9.
- [15] Bolumar T, Bindrich U, Toepfl S, Toldrá F, Heinz V. Effect of electrohydraulic shockwave treatment on tenderness, muscle cathepsin and peptidase activities and microstructure of beef loin steaks from Holstein young bulls. Meat Science. 2014;98:759-65
Contact:
Simone Schiller, Managing Director of the DLG Competence Centre Food, Frankfurt am Main, S.Schiller@DLG.org