Extrusion – Traditional structuring and texturizing processes for the food production of the future
DLG Expert report 2/2022
Authors:
Dr. Christoph Verheyen, Johanna Jorkowski, Dr. Anna Martin
Fraunhofer-Institut für Verfahrenstechnik und Verpackung IVV
christoph.verheyen@ivv.fraunhofer.de
Content
- 1. Extrusion - Texturizing of foods with tradition
- 2. Extrusiondesign – Screws and dies as most significant structural components in the extruder
- 3. Extrusion - temperature and water content define the process
- 4. Meat substitutes - protein source and functionality define the texture
- 5. Post-processing – refinement of the extrudates
- 6. Outlook - extrusion an important building block in the non-animal food supply
- Literature:
- contakt
1. Extrusion - Texturizing of foods with tradition
Extrusion is a versatile and efficient food processing technique that is used for the preparation of mostly starch and protein based feed and food products. Extrusion is a process in which a material or material mixture is forced through an opening or a die with a defined shape. The thrust required is generated mechanically through the pressure of a moving piston or the rotation of one or more conveying screws.
The products manufactured by extrusion are generally referred to as “extrudates”. The origin of the process lies in the forming or shaping of materials and was first used in 1797 in England to produce seamless lead pipes. In the 1930s, the first heated screw press was used in the plastics industry. Simultaneously, this principle of screw extrusion also replaced piston extrusion in the food sector, which had previously been used for first batch applications in meat and sausage processing. Single screw extrusion was first applied to pasta production, revolutionising the food industry as the increased throughput and high functionality of a screw extruder made continuous and trouble-free pasta production possible [1]. Later in the 1960s, twin screw extruders were introduced, which allowed impressive product diversification, not least due to their high flexibility in terms of screw design. In the 1980s, this was reflected in a wide range of new snack and cereal products. However, further innovative product categories were subsequently a long time coming, despite the fact that defatted soy flours were processed into dry-textured soy proteins (TVP = textured vegetable protein) by thermoplastic cooking extrusion as early as the 1960s, making them the first meat alternatives available in Germany [2].
Due to oecological and ethical motives to reduce consumption of animal products, without sacrificing familiar taste and haptics, a more than 200 years old process developed into one of the most promising texturizing processes of non-animal raw materials.
2. Extrusiondesign – Screws and dies as most significant structural components in the extruder
In general, three extruder classes for use in the food industry can be differentiated: Piston, roller and screw extruders. Screw extruders are most commonly used and can be categorized into single- and twin-screw extruder. In screw extruders, effective screw design is of utmost importance for alignment and optimization of the extrusion process. The screw or shaft performs a variety of tasks during the extrusion process, strongly dependent on the design. The main characteristics of an extrusion screw are length, ratio of length to diameter (L/D ratio), the respective lengths of the feed, compression and discharge zones, the flight width, the flight pitch and the flight depths in each section. Especially the (L/D ratio) is an important attribute that specifies the screw length (L) as a multiple of the screw diameter (D). The compression ratio plays an equally important role. This ratio is calculated from the depths of the inlet and outlet zones, whereby a ratio of 1:1 corresponds to no compression and a ratio of 1:5, for instance, corresponds to strong compression [3]. The length designation of screw extruders is given as 30D, for example, and means that the length is 30 times the screw diameter. All operations that can be influenced by residence time or speed (such as the melting and mixing of polymers) can be specifically controlled via the screw length. In the first stage of the extrusion process the raw materials are dosed by means of gravimetric/volumetric metering devices (dry components) or by means of pumps (liquid components) and drawn in by the screw.
The material is mechanically stressed and mixed by the rotation of the extruder screws. This can lead to high local shear rated and thus to the generation of frictional heat. Accordingly the material is subjected to high mechanical and thermal stress, resulting in a so-called “melt”. Furthermore, high pressure is build up through steep screw pitches or a significant flow channel taper between the housing and die area. The geometry of the screws, which often changes during the process length, impacts the throughput, the mixing efficiency, the melting rate and the local distribution of the material temperature directly [4]. Pitch and flight depth are essential for dimensioning the screw or single screw elements, since those parameters, in combination with the filling degree, define the pressure needed to flow through the screw area over the entire process length and furthermore influence the structure of the extrudate [5]. If all other process parameters are constant, the residence time in the screw is directly proportional to its length and inversely proportional to the rotation speed. Depending on the type of the extruder one or two screws are rotating in the extruder housing.
The design of process- and product-optimised screws is an important factor for the machine design and defines the process efficiency and quality of the extrudates. Usually, barrels with smooth inner surfaces are used, as a coating would constitute the risk of sticking or rotating along the screw. Typical design elements inside the barrels are longitudinal (axial groove) or spiral grooves that enable a higher throughput, better barrel mixing or an improved shearing effect [4]. Simultaneously, a reverse transport counteracts the optimal product transportation and an exceeding of the tolerable maximum pressure at the die outlet can occur. Active zone-temperature control of the screw area can be facilitated through a double jacket using cooling and heating units. Depending on the design, physicochemical modification processes of the material can be controlled via a varying number of temperature zones along the housing in combination with pressure and temperature sensors.
At the end of the screw area, the final shaping of the product takes place in the so-called die area. The geometry and design of the die are critical variables for the visual appearance (size, shape) as well as for substantial sensory properties (texture) of the products.
Furthermore, the die area defines the degree of filling, the pressure as well as the temperature profile in the process chamber of the extruder. The product leaves the die as a continuous strand which can be cut into pieces of any length by a pelletising unit, for example in the form of rotating knives. Solid pellets, fibrous flakes or strongly puffed products can be produced with an extruder, depending on the temperature, pressure and shape of the strands at the die outlet. Classic starch-based extrusion products, such as flat breads or snacks, which lose large amounts of water vapour due to the high pressure drop at the die outlet, are contrasted by new fibrous meat-like products. These products are produced under adapted process conditions using a special cooling die that cools the extrudates to approx. 40 – 50 °C before exiting the die, wherefore neither evaporating water nor strong expansion effects occur [6].
Single-screw extruders
The abovementioned classic extrusion products, such as breakfast cereals, snacks or pasta, are mainly produced using single-screw extruders. These are the simplest extrusion systems in terms of design and are associated with low investment costs. Here, the material mixture is moved by a single screw through the extrusion barrel towards the die. A standard single-stage extruder screw is divided lengthwise into feed zone L1 (feeding), compression zone L2 (compression) and metering zone L3 [8]. In the feed zone, free-flowing dry materials are fed into the extruder screw and transported towards zone L2. The feed zone is usually actively cooled and has deeper screw flights to avoid agglomeration. In the following compression zone L2, the compaction of the mass is further accelerated.
At the same time, the energy input into the product matrix is increased by increasing pressure and temperature as well as increasing shear. Furthermore, the product moisture can be increased by direct injection of water or water vapour, so that particles agglomerate and biopolymers such as starch or proteins gelatinise or begin to unfold. In the cooking zone, where the pressure, temperature as well as the mixing and shearing effect are highest, the homogeneous, compacted mass is conveyed to the die [5]. This is where, due to high local shear stresses and temperatures, the final texturing takes place as a consequence of starch gelatinisation and denaturation of the proteins. The resulting products can be fully cooked, low density cereal snack products or partially cooked, moulded pasta products with high density.
Twin screw extruder
Twin-screw extruders allow greater flexibility in processing liquid, sticky and lipid raw materials that would not experience friction and shear forces in a single-screw extruder.The same applies to raw material mixtures that exhibit an inhomogeneous particle size distribution. In extrusion cooking, it is therefore common practice to work with more expensive twin-screw extruders. Both screws, which run in a barrel with an eight-shaped bore and intermesh depending on the distance between them (intermeshing screws), can work in the same or opposite direction and thus provide different pumping effects or die pressures. The rotating screws are constructed modular from conveying, kneading or return elements, depending on the desired mixing efficiency and product type [9]. In co-rotating twin-screw extruders, the direction and speed of both screws are identical.
In addition, the screws must have the same geometry. Advantages of co-rotating twin-screw extruders are the uniform product transport, the independence of the residence time from the screw rotation speed, a narrow residence time distribution, effective heat transfer and the high self-cleaning effect of the process chamber [4]. Counter-rotating screws are characterised by very good conveying properties, but are usually made from a single piece. Modular adaptation to different process targets is therefore not possible. Hence, they are often used in monoproduct lines and for products such as chewing gum, which, due to their material properties, cause long residence times and low throughputs.
3. Extrusion - temperature and water content define the process
Extrusion enables a wide range of applications in the food and feed industry. This is due to both differences in the designs described above as well as to the large number of multivariate independent process variables. Relevant are, for example, screw speed, feed rate, die geometry, feed rate, residence time, energy input (mechanical and thermal) and pressure development [10]. A targeted control of process parameters in combination with the selection of raw materials creates a wide range of process conditions that allow an almost limitless spectrum of extruded products. According to the temperature, these processes are classified into cold and cooking extrusion. A further differentiation of the cooking extrusion is made based on the water content in the melt: low and high moisture extrusion.
Cold extrusion
The cold extrusion process typically takes place at temperatures between 40 – 75 °C in order to minimise heat-induced processes such as starch gelatinisation, protein denaturation or browning reactions [11]. Therefore, the process chamber is usually not actively heated, but actively cooled to compensate for the frictional heat that arises. The pressure in the process chamber lies between 6 – 10 bar. Cold extrusion is mainly used in dough and confectionery technology and requires a high water content at low screw speed. Typical products are sweets as well as doughs and pasta.
Cooking extrusion
Cooking or hot extrusion takes place at temperatures between 130 - 180 °C and, on an industrial scale, at a pressure range of 120 – 250 bar. Cooking extrusion can be described as a continuous high-temperature short-time process in which viscous hardenable materials are pressed through a forming die [12]. In addition to classical applications such as the production of starch-based puffed cereal snack and breakfast products, cooking extrusion can be used to modify plant storage proteins in a targeted manner so that product structures similar to animal muscle fibres are generated [13]. Under the influence of mechanical and thermal energy, the typically powdery raw materials are plasticised with water, cooked and shaped into the desired product form through a die.
Hereby, the water content is a critical parameter, as it has a defined influence on the viscosity of the product melt, the heat transfer between barrel and product, as well as on critical physicochemical processes such as gelatinisation or melting temperature [14]. Therefore a classification of individual cooking extrusion processes into low moisture extrusion (< 40 % product moisture) and high moisture extrusion (> 40 % product moisture) is based on the water content.
Low moisture extrusion
Low moisture extrusion, (moisture content < 40 %) can be used to produce expanded or fine-fibre extrudates, depending on the setting of the extrusion parameters, the process management and recipe design. Typical examples are pelletised animal feed, cereals, pasta, snack products, confectionery or meat analogues such as minced meat substitutes [15]. Due to the high mechanical shear of the highly viscous masses, partial biopolymer cooking is already initiated in the process chamber and the product matrix expands shortly after die exit [16].
Prerequisite for texturisation is exceeding the melting and glass transition temperature, which serves to generate a flowable melt and allows it to exit the die [17]. The expansion of the product is promoted by a short die at the end of the process chamber, as this narrows the flow cross-section and generates a high material pressure build-up just before the die exit. After exiting the die, the sudden pressure-drop causes the superheated water to evaporate abruptly and the formation of water vapour bubbles causes the product matrix to expand (flash-off). Subsequently, the hot mass relaxes and cools down simultaneously [16]. A stabilisation of the vapour bubbles and thus preservation of the porous product structure requires the glass transition temperature to be underwent as quickly as possible. This causes a solidification of the product structure and counteracts a regression of the expansion by elastic recovery.
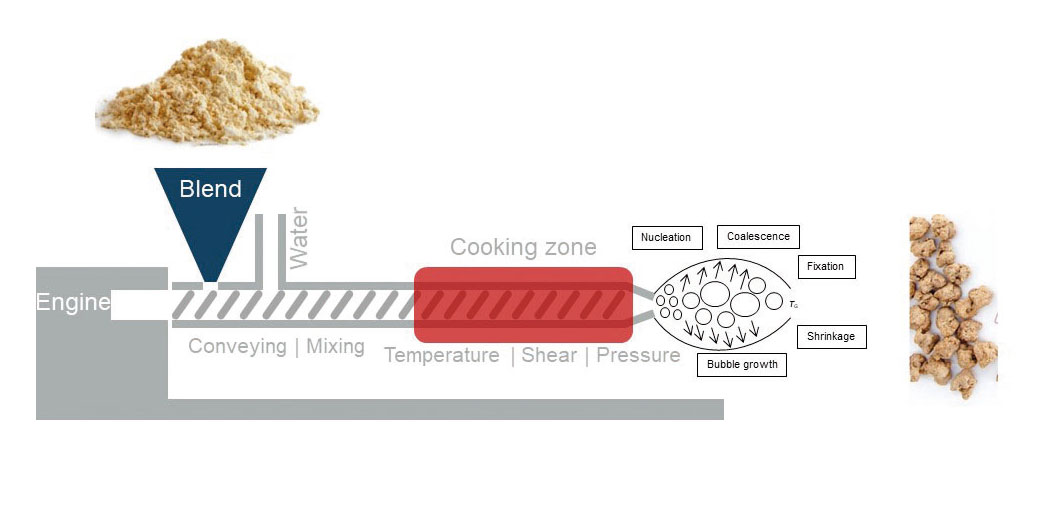
High moisture extrusion
In high moisture extrusion, mainly protein masses with water contents > 40 % are cooked. After reaching very high temperatures in the extruder housing, the hot melt is conveyed through a long cooling die with temperatures < 80 °C. This leads to the formation of an anisotropic, i.e. aligned product texture, which is formed by an axial flow profile of the two-phase system protein and water [20]. The resulting plant intermediates can be processed further, e.g. by breading or marinating, to produce so-called “one-piece” meat substitutes (Schnitzel, steak). In principle, there are hardly any limitations regarding the application of the produced textures, so that product categories such as vegan nuggets, gyros, meatballs etc. can be produced.
4. Meat substitutes - protein source and functionality define the texture
Even though the product properties can be strongly influenced by adjusting the process parameters during extrusion, the material properties are particularly decisive for the product-typical textures of the extrudates. Different functional raw material properties lead to significant differences in the product structures. The main components of fibrous meat analogues are vegetable proteins (20 – 50 %), vegetable lipids (0 – 5 %), polysaccharides (2 – 30 %), as well as other ingredients that approximate the texture, appearance and consistency to meat (see Table 1).
Table 1: Main ingredients for the production of meat substitutes [21]
Ingredient | Source | Main function |
Non-animal proteins | Plant-based: soy, wheat, pulses, peas, lupines, rice and potatoes other: microalgae, seaweed, insects | Nutrition, structure, colour, texture and technofunctionality |
Lipids | Coconut oil, cocoa butter, sunflower oil, rapeseed oil, sesame oil fat replacements: oleo gels and fibres | Taste, texture and mouthfeel |
Polysaccharides | Native starches, flours, dietary fibres, fibres | Consistency and waterbinding |
Flavours | Yeast extract, paprika, sugar, spices and herbs | Flavour |
Colourants | Lycopin, beet juice extract and leghaemoglobin | Imitation of meat colour |
Additives | Tocopherols, zinc gluconate, thiamine hydrochloride, Sodium, ascorbic acid, vitamin B12 | Nutrirional improvement |
Since plant proteins differ in terms of functionality, composition and nutritional value depending on the source, variety, cultivation conditions, pre-processing and purity, proteins from one or more sources are used, depending on the product and desired functionality [22]. Soy protein is the most commonly used protein to date, as it is highly suitable for meat substitutes due to its beneficial functional properties and balanced amino acid profile [23, 24]. Despite low costs and advantageous technofunctional properties, almost three quarters of the globally cultivated soy beans are genetically modified and thus are becoming unpopular raw materials for an increasing number of manufacturers and consumers [25].
Wheat protein alias gluten is, due to its viscoelastic properties, also a very suitable protein for the production of meat substitutes, as it favours the formation of fibre-like structures due to its high reactivity and high cysteine content [26, 27]. Moreover, gluten can act as a binding agent in combination with proteins and minimise cooking losses during processing, so that the final extrudates exhibit a cohesive structure [26].
Pea protein is a valued alternative to soy protein due to its high adaptability. Due to its hypoallergenicity and favourable functional properties, it is mostly used in combination with other raw materials such as gluten. Thus, pea protein can be used to optimise nutritional and structural properties [28]. Especially for the formulation of gluten-free meat alternatives, proteins from rice, potatoes, legumes, oilseeds or pseudocereals are a good alternative. However, material properties and fields of application have not yet been sufficiently investigated. The same applies to innovative raw material sources such as microalgae, fungi and insects that exhibit high protein contents and very good nutritional values and can, in the long term, be produced in a cost-effective and sustainable way [29, 30, 30].
5. Post-processing – refinement of the extrudates
Protein-based extrusion does not necessarily lead to the production of final product structures. Regularly, by the application of refinement steps intermediates are upgraded to products with optimal sensory and textural properties.
In so-called post-processing, one or more process steps are used that are, like the equipment used, supplanted from the butchery sector. These include mincing, marinating, breading or coating, mixing and shaping. The last two process steps in particular are essential to ensure that the substitute products are as identical as possible to the animal “models” in terms of texture and appearance.
In this context, mixing the extrudates with other ingredients is essential. An important group of ingredients are lipids, which are either rich in saturated fatty acids (e.g. coconut oil or cocoa butter) or unsaturated fatty acids (e.g. sunflower oil or rapeseed oil) and characterise the texture and mouthfeel [32-35]. Furthermore, the source and composition of the fatty acids are crucial, as they contribute to a meat-like taste through lipid oxidation and Maillard reaction products [36].
Polysaccharides play an important functional and structural role in the formation of meat surrogates, as they contribute decisively to the consistency and cohesion of the products, due to their thickening and emulsifying properties [34, 37, 38]. Native starches from e.g. wheat or potato are mainly used as fillers to improve texture and consistency [24, 39]. The same applies to fibres derived from various sources such as e.g. pea or soy. The most prominent and commonly used fibre is methylcellulose, which, like cellulose, cannot be metabolised by the human organism and is thus considered a dietary fibre. Other hydrocolloids that improve water binding while reducing cooking loss are e.g. carrageenan, xanthan or alginate [71].
To improve the flavour profile or mask unfavourable aroma components of legume proteins, flavour-enhancing ingredients such as yeast extract, spices or other naturally and synthetically produced flavourings are commonly used. The imitation of the red meat colour is achieved by the addition of colouring agents such as lycopene, beetroot juice concentrate or leghaemoglobin from soya roots. The imitation of white meat colour in poultry or fish substitutes can be achieved by titanium dioxide [28, 40-42].
By adding ascorbic acid or polyphenols, the added colour pigments can be stabilised during heating [28, 43]. Optionally, ingredients such as vitamin B12, tocopherols, zinc, gluconate or niancin can be added, which are typically consumed in animal-based diets and thus improve the nutritive value of meat substitutes.
6. Outlook - extrusion an important building block in the non-animal food supply
One of the challenges of a sustainable future food supply is the reduction of the high meat consumption in industrial countries. Traditional plant protein products from soy or wheat protein, such as tofu, tempeh or seitan, which are an integral part of the diet in Asian countries, are associated with a significant loss in culinary indulgence around here. The reasons are especially the plant-like flavour and textures atypical for meat. Hence, the globular plant storage proteins have to be processed into a fibrillar form to provide sustainable, protein-rich and sensorially optimized alternative products with meat-like characteristics. It is remarkable that this transformation process was implemented with a process that has a history of more than 200 years and yet constitutes one of the greatest innovations of the last decade in the food industry.
The texturisation of non-animal protein sources to meat-analogue products, which do not differ from meat-containing products in terms of texture, mouthfeel, taste and nutritional value may only be the beginning. Because in addition to new raw material sources, novel processes, eg. wet- and electro-spinning, bioprinting or cellular agriculture, hold the potential to develop new, animal-free products matrices with entirely new textures.
Literature:
[1] Ainsworth, P. (2011). Extrusion. Food processing handbook, 429-453.
[2] Akdogan, H. (1999). High moisture food extrusion. International journal of food science & technology, 34(3), 195-207.
[3] Giles Jr, H. F., Mount III, E. M., & Wagner Jr, J. R. (2004). Extrusion: the definitive processing guide and handbook. William Andrew.
[4] Riaz, M. N. (2000). Practical considerations in extrusion processing. In Extruders in food applications (pp. 149-165).
CRC Press.
[5] Fattmann, G., Greif, H., Limper, A., & Seibel, S. (2004). Technologie der Extrusion: Lern-und Arbeitsbuch für die Aus-und Weiterbildung.
[6] Mercier, C., Linko, P., & Harper, J. M. (1989). Extrusion cooking.
[7] Pietsch, V. L., Schöffel, F., Rädle, M., Karbstein, H. P., & Emin, M. A. (2019). High moisture extrusion of wheat gluten: Modeling of the polymerization behavior in the screw section of the extrusion process. Journal of Food Engineering, 246, 67-74.
[8] Hansen, F., Knapie, W., & Potente, H. (1989). Handbuch der Kunststoff-Extrusionstechnik. I Grundlagen. Carl Hanser Verlag, München-Wien.
[9] Koch, L., Emin, M. A., & Schuchmann, H. P. (2017). Influence of processing conditions on the formation of whey protein-citrus pectin conjugates in extrusion. Journal of Food Engineering, 193, 1-9.
[10]. Harper, J. M. (2019). Extrusion of foods. CRC press.
[11] Zitzmann, K. (1982): Die Extrusion als Möglichkeit der Verarbeitung von Nichtbackweizen und Backweizen zu Nahrungsmitteln. Diplomarbeit. Humboldt Universität zu Berlin.
[12] Schuchmann, H. P. (2008). Extrusion zur Gestaltung von Lebensmittelstrukturen. Chemie Ingenieur Technik, 80(8), 1097-1106
[13] Osen, R., & Agulla, K. (2019). Die Veggie-Generation 2.0. Neue Proteinzutaten und Verarbeitungstechnologien für schmackhafte Produkte. Fleischwirtschaft. 98 (2019), 3, 84-88.
[14] Schuchmann, H. P., & Schuchmann, H. (2012). Lebensmittelverfahrenstechnik. John Wiley & Sons.
[15] Philipp, C., Emin, M. A., Buckow, R., Silcock, P., & Oey, I. (2018). Pea protein-fortified extruded snacks: Linking melt viscosity and glass transition temperature with expansion behaviour. Journal of Food Engineering, 217, 93-100.
[16] Moraru, C. I., & Kokini, J. L. (2003). Nucleation and expansion during extrusion and microwave heating of cereal foods. Comprehensive reviews in food science and food safety, 2(4), 147-165.
[17] Shrivastava, A. (2018). Introduction to plastics engineering. William Andrew.
[18] Horvat, M., Emin, M. A., Hochstein, B., Willenbacher, N., & Schuchmann, H. P. (2013). A multiple-step slit die rheometer for rheological characterization of extruded starch melts. Journal of Food Engineering, 116(2), 398-403.
[19] Martin, A., Osen, R., Karbstein, H. P., & Emin, M. A. (2021). Impact of rapeseed press cake on the rheological properties and expansion dynamics of extruded maize starch. Foods, 10(3), 616.
[20] Benbow, J., & Bridgwater, J. (1993). Paste flow and extrusion.
[21] Fu-Hung Hsieh, Harold E. Huff (2011) Meat Analog Compositions and Process. US20120093994A1
[22] Hoehnel, A., Axel, C., Bez, J., Arendt, E. K., & Zannini, E. (2019). Comparative analysis of plant-based high-protein ingredients and their impact on quality of high-protein bread. Journal of Cereal Science, 89, 102816.
[23] Kumar, P., Chatli, M. K., Mehta, N., Singh, P., Malav, O. P., & Verma, A. K. (2017). Meat analogues: Health promising sustainable meat substitutes. Critical reviews in food science and nutrition, 57(5), 923-932.
[24] Yuliarti, O., Kovis, T. J. K., & Yi, N. J. (2021). Structuring the meat analogue by using plant-based derived composites. Journal of food engineering, 288, 110138.
[25] Mintel (2019) The meat substitute ingredients to watch - Mintel
[26] Chiang, J. H., Loveday, S. M., Hardacre, A. K., & Parker, M. E. (2019). Effects of soy protein to wheat gluten ratio on the physicochemical properties of extruded meat analogues. Food Structure, 19, 100102.
[27] Samard, S., Gu, B. Y., & Ryu, G. H. (2019). Effects of extrusion types, screw speed and addition of wheat gluten on physicochemical characteristics and cooking stability of meat analogues. Journal of the Science of Food and Agriculture, 99(11), 4922-4931.
[28] Schreuders, F. K., Dekkers, B. L., Bodnár, I., Erni, P., Boom, R. M., & van der Goot, A. J. (2019). Comparing structuring potential of pea and soy protein with gluten for meat analogue preparation. Journal of Food Engineering, 261, 32-39.
[29] Palanisamy, M., Töpfl, S., Berger, R. G., & Hertel, C. (2019). Physico-chemical and nutritional properties of meat analogues based on Spirulina/lupin protein mixtures. European Food Research and Technology, 245(9), 1889-1898.
[30] Caporgno, M. P., Böcker, L., Müssner, C., Stirnemann, E., Haberkorn, I., Adelmann, H., ... & Mathys, A. (2020). Extruded meat analogues based on yellow, heterotrophically cultivated Auxenochlorella protothecoides microalgae. Innovative Food Science & Emerging Technologies, 59, 102275.
[31] Stephan, A., Ahlborn, J., Zajul, M., & Zorn, H. (2018). Edible mushroom mycelia of Pleurotus sapidus as novel protein sources in a vegan boiled sausage analog system: functionality and sensory tests in comparison to commercial proteins and meat sausages. European Food Research and Technology, 244(5), 913-924.
[32] Dekkers, B. L., Emin, M. A., Boom, R. M., & van der Goot, A. J. (2018). The phase properties of soy protein and wheat gluten in a blend for fibrous structure formation. Food Hydrocolloids, 79, 273-281.
[33] Pietsch, V. L., Bühler, J. M., Karbstein, H. P., & Emin, M. A. (2019). High moisture extrusion of soy protein concentrate: Influence of thermomechanical treatment on protein-protein interactions and rheological properties. Journal of Food Engineering, 251, 11-18.
[34] Lin, S., Huff, H. E., & Hsieh, F. (2000). Texture and chemical characteristics of soy protein meat analog extruded at high moisture. Journal of Food Science, 65(2), 264-269.
[35] Emin, M. A., Quevedo, M., Wilhelm, M., & Karbstein, H. P. (2017). Analysis of the reaction behavior of highly concentrated plant proteins in extrusion-like conditions. Innovative Food Science & Emerging Technologies, 44, 15-20.
[36] Diez-Simon, C., Mumm, R., & Hall, R. D. (2019). Mass spectrometry-based metabolomics of volatiles as a new tool for understanding aroma and flavour chemistry in processed food products. Metabolomics, 15(3), 1-20.
[37] Lin, S., Huff, H. E., & Hsieh, F. (2002). Extrusion process parameters, sensory characteristics, and structural properties of a high moisture soy protein meat analog. Journal of Food Science, 67(3), 1066-1072.
[38] Yao, G., Liu, K. S., & Hsieh, F. (2004). A new method for characterizing fiber formation in meat analogs during high‐moisture extrusion. Journal of Food Science, 69(7), 303-307.
[39] Krintiras, G. A., Göbel, J., Van der Goot, A. J., & Stefanidis, G. D. (2015). Production of structured soy-based meat analogues using simple shear and heat in a Couette Cell. Journal of Food Engineering, 160, 34-41.
[40] Fraser, R. Z., Shitut, M., Agrawal, P., Mendes, O., & Klapholz, S. (2018). Safety evaluation of soy leghemoglobin protein preparation derived from Pichia pastoris, intended for use as a flavor catalyst in plant-based meat. International Journal of Toxicology, 37(3), 241-262.
[41] Oreopoulou, V., & Tzia, C. (2007). Utilization of plant by-products for the recovery of proteins, dietary fibers, antioxidants, and colorants. In Utilization of by-products and treatment of waste in the food industry (pp. 209-232). Springer, Boston, MA.
[42] Rayner, M. G., Rayner, J. L., & Miller, R. (2018). U.S. Patent No. 9,968,115. Washington, DC: U.S. Patent and Trademark Office.
[43] Trottet, G., Fernandes, S., Grunz, G., Thoma, J. P., & Brunner-Komorek, K. (2018). U.S. Patent Application No. 15/558,344.
Contact
Managing director
Simone Schiller • DLG Competence Center Food, Location Frankfurt • Tel: +49(0)69/24 788-390 S.Schiller@DLG.org